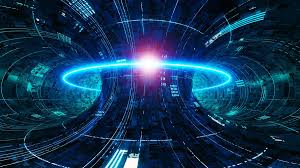
Author: Preethika Arunkumar
When most of the general public thinks about nuclear weapons, we think about the massive, destructive weapons forming vast clouds of radiation that the U.S. tested during the Cold War period. What people most often picture is the effect of a huge energy release due to nuclear fission. Fission is caused by the splitting of a heavy nucleus once it is hit by a neutron, forming lighter nuclei and more neutrons, which proceed to hit more heavy nuclei. This creates a chain reaction that occurs until the nucleus reaches a stable, less massive state. Fission, though once used to create nuclear weapons, is now used in the controlled environment of a fission reactor to produce energy.
However, there is another type of nuclear reaction, known as fusion. Fusion occurs when light nuclei merge to form a heavy one. It, along with fission, was used in the creation of destructive weapons, namely hydrogen bombs. However, fusion has not yet been tamed enough to form a reliable source of energy, but scientists have high hopes for this method of reaction because it is “safer” and is a potentially unlimited source of energy.
Nuclear fusion primarily occurs in stars and is their main source of energy. Stars, which are essentially giant orbs of hot gases, contain hydrogen gas, which is squeezed and heated due to the immense pressures at the star’s core. These are prime conditions for the nuclear fusion of hydrogen, which combine to form helium and eventually heavier substances. Gravity causes crushing forces that lead to the star’s collapse, which initiates fusion reactions that release energy, expanding the star once again. For this reason, a star’s life exists in a perfect yet precarious balance, for the most part.
Nuclear fusion can release large quantities of energy, far greater than that which can be produced by nuclear fission. But why does the merging of small atoms produce more energy than the breaking apart of larger ones? To better visualize this, picture a valley steep on the left and shallower on the right. The left side represents elements with low atomic mass; the right side, high mass. Iron is at the valley’s lowest point. Light elements like hydrogen “roll down” a steep slope through fusion, releasing great energy. Heavy elements like uranium “roll down” a gentle slope through fission. The steeper the slope, the more energy is released.
To harness fusion energy, scientists have developed several innovative methods. Fusion fuel exists in a state called plasma—extremely hot, with electrons separated from nuclei. Plasma cannot be contained in a normal container, so scientists use magnetic fields. Devices like tokamaks, spheromaks, and stellarators use strong magnetic fields in donut-shaped chambers to keep plasma suspended and prevent it from touching the walls.
Another approach uses lasers. In inertial confinement fusion, intense lasers target a tiny pellet of fuel, compressing and heating it to trigger fusion. The National Ignition Facility (NIF) in the U.S. is a leader in this area, using 192 powerful laser beams. Yet another method, the pinch method, sends electric currents through plasma to generate magnetic fields that compress and heat it for fusion. Additional experiments include particle accelerators and piston-based compression systems.
Despite the diversity of devices, fusion has been notoriously difficult to sustain. Scientists aim to reach “breakeven”—where the energy output from fusion exceeds the energy put in. Many inventors have attempted this, sometimes prematurely claiming success. A notable incident was the infamous cold fusion debacle.
In 1989, chemists Stanley Pons and Martin Fleischmann claimed to achieve fusion at room temperature using heavy water and palladium. They observed excess heat and assumed fusion occurred. However, their results were inconsistent, and neutron testing failed to support their claims. Despite this, they rushed to publish and publicize their findings before peer verification. They skipped key scientific steps, like control groups and transparent methods. The fallout from their unverified claim damaged trust in cold fusion research.
Many scientists have since made bold claims about breakeven, only to fall short under scrutiny. But then came December 2022. On December 13, the NIF announced it had achieved scientific breakeven with laser fusion—verified by five independent teams. Though this result is promising, skepticism remains essential, given fusion’s rocky past.
Even if the NIF’s success holds up, widespread use of fusion energy is still a distant goal. Challenges include scaling up, achieving consistent energy output, and cost-efficiency. NIF’s lasers consume more energy than the fusion reaction releases overall, and they damage themselves with each shot. Energy conversion and commercial viability remain unresolved issues.
Fusion reactions involving deuterium and tritium are considered most promising. But tritium is rare and must be generated in fission reactors, creating dependency. Tritium’s short half-life and limited recoverability compound the problem. Fusion also produces radiation—neutrons and particles that damage reactor walls. These must be frequently replaced and safely disposed of, adding to the cost and complexity. Fusion waste is less radioactive than fission’s, but produced in much greater volume.
Though we may have reached a milestone with breakeven, fusion energy still requires massive effort before becoming viable. It is often seen as superior to fission, but it comes with its own serious drawbacks. Global cooperation, funding, and patience will be needed. And as the race toward practical fusion continues, we must remain vigilant—ensuring science leads the way, not premature promises.
Works Cited
- Anomalous Neutrons - Understanding Science, 2022
- Approaches to Fusion – US Fusion Energy
- “Fusion 'Breakthrough' Won’t Lead to Practical Fusion Energy” – IEEE Spectrum
- Jassby, Daniel. “Fusion Reactors: Not What They’re Cracked up to Be.” – Bulletin of the Atomic Scientists
- NASA. “Star Basics.”
- Seife, Charles. Sun in a Bottle. Penguin Group, 2009.
- “What Is the Difference between Nuclear Fission and Fusion?” – Encyclopedia Britannica
- Yirka, Bob, and Phys.org. “NIF Fusion Breakeven Claims Peer Reviewed and Verified by Multiple Teams.”